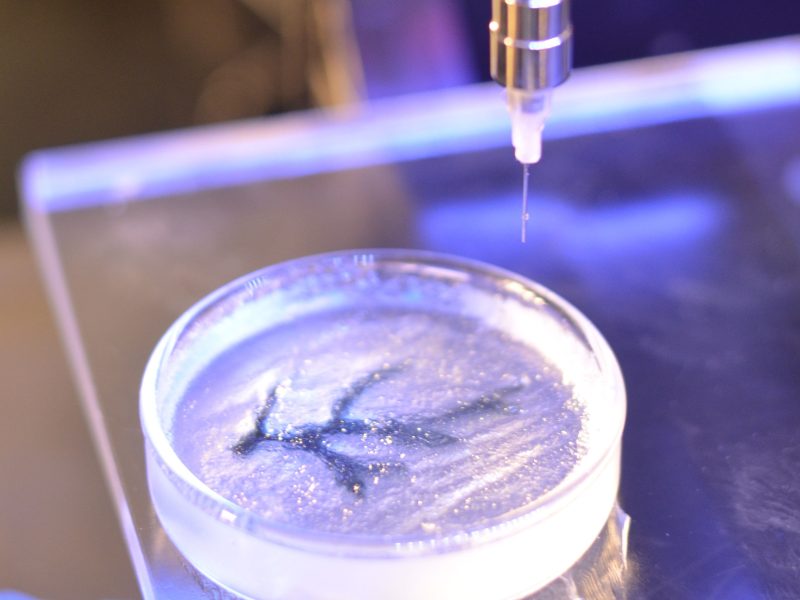
3D Bioprinting with Cell-Laden Bioinks: Innovations in Photoinitiated Crosslinking for Enhanced Cytocompatibility
1.Introduction
The field of 3D bioprinting has undergone tremendous advancements over the past decade, presenting novel opportunities in tissue engineering, regenerative medicine, and even organ fabrication. As a technique that involves the precise layering of biological materials, 3D bioprinting enables the creation of complex tissue structures that mimic the architecture and function of natural tissues. However, the success of this technology heavily relies on the compatibility of the materials and processes used, particularly when it comes to bioinks laden with live cells. Bioinks must not only be biocompatible but also have the necessary mechanical properties to support tissue growth, while still being printable.
One of the most promising approaches within 3D bioprinting is light-based bioprinting, where light energy is employed to solidify liquid bioinks into functional tissue structures. Light-based bioprinting offers superior speed and precision compared to other techniques, such as extrusion or inkjet bioprinting, which rely on mechanical forces. However, the use of ultraviolet (UV) and violet light sources in these processes poses challenges in terms of cytocompatibility, as UV exposure can damage cells and DNA, leading to low cell viability.
To overcome this, the development of new photoinitiators and bioinks that can be activated by safer, visible-light sources, such as green or red light, has become a focal point of research. This essay explores the latest advancements in 3D bioprinting using biosafety photoinitiators, emphasizing the use of green-to-red light sources for hydrogel crosslinking and successful cell encapsulation. We will also discuss the implications of these developments for tissue engineering and the future of bioprinting technologies.
2.The Role of Light-Based 3D Bioprinting in Tissue Fabrication
3D bioprinting has quickly become one of the most exciting and versatile methods for creating biologically relevant structures. The capacity to engineer complex tissue geometries with high precision makes it an invaluable tool in areas such as drug testing, personalized medicine, and the potential creation of transplantable organs. Light-based 3D bioprinting, in particular, holds several advantages over other methods due to its ability to rapidly cure and solidify bioinks using light, enabling high-speed fabrication of detailed structures.
In light-based bioprinting, photocurable bioinks containing cells and biomaterials are subjected to controlled doses of light, which triggers crosslinking reactions within the bioink. This results in the formation of stable, 3D hydrogel structures that can encapsulate living cells in a biocompatible matrix. One of the most widely used materials in this context is gelatin methacrylate (GelMA), a derivative of natural gelatin that can be photocrosslinked to form hydrogels.
While light-based 3D bioprinting offers unique advantages such as superior print resolution and enhanced spatial control, the cytotoxicity of UV and violet light poses a significant challenge. UV light exposure has been shown to induce DNA damage and apoptosis in cells, which undermines the viability of the printed tissue constructs. Therefore, there is a growing interest in developing visible-light-driven bioprinting systems that can offer the same level of precision while minimizing cellular damage.
3.Challenges in UV-Violet Light-Based Bioprinting
UV and violet light have traditionally been used in light-based 3D bioprinting because they allow for fast and efficient crosslinking of photoreactive bioinks. Photoinitiators, such as TPO-L, lithium phenyl(2,4,6-trimethylbenzoyl)phosphinate (LAP), and Irgacure 2959, are commonly used in combination with UV light to trigger free radical polymerization, which results in the rapid formation of hydrogels. The fast curing times provided by UV light are particularly advantageous in applications requiring high precision and complex geometries, such as in the creation of vascular structures.
However, despite these advantages, the use of UV light comes with significant drawbacks. UV light is known to be harmful to living cells, causing oxidative stress, DNA damage, and other deleterious effects that can compromise cell viability. As a result, tissues printed with UV-light-cured bioinks may suffer from reduced functionality and structural integrity, especially in the context of long-term applications like regenerative therapy or tissue grafting.
To mitigate the cytotoxic effects of UV light, researchers have explored alternative methods for photocuring bioinks. One promising approach involves expanding the wavelength of light used in bioprinting to include visible light (400–780 nm) and near-infrared (NIR) light (λ ≥ 780 nm), which are less harmful to cells. By using these gentler light sources, it is possible to achieve photocuring with reduced cellular damage, thus improving the overall viability and functionality of the printed tissues.
4.Visible Light Photopolymerization for Cytocompatibility
The transition from UV to visible light for bioprinting presents several challenges, particularly in terms of developing new photoinitiators that are compatible with visible light. Traditional photoinitiators, such as Irgacure 2959, are optimized for UV wavelengths and are not effective under visible light. To address this issue, researchers have developed multicomponent photosystems that function via a Type II reaction mechanism, which involves a photosensitizing dye and a co-initiator molecule. These systems facilitate rapid photocuring under visible light by enabling photoinduced electron transfer (PET) processes between the dye and the co-initiator.
Recent advances in the development of visible-light photoinitiators have opened up new possibilities for bioprinting with improved cytocompatibility. For example, Lynn et al. successfully employed a near-infrared sensitizer (H-Nu 815) for digital light processing (DLP) bioprinting, achieving high-resolution structures with minimal cellular damage. Similarly, up-conversion particles have been used to enable photocuring with NIR light, offering another alternative to traditional UV-based systems.
Despite these advances, the development of visible-light photoinitiators remains a relatively nascent field, and many of the available options exhibit suboptimal cytocompatibility. Moreover, the variety of visible-light-activated initiators is still limited, making it difficult to achieve the same level of performance as UV-based systems. As a result, there is a pressing need for the development of new photoinitiators that can operate under visible light while maintaining high levels of cell viability.
5.Novel Photoinitiators for Green-Red LED Crosslinking
In an effort to address the limitations of existing photoinitiators, researchers have focused on the design of novel photoinitiators that can be activated by green-to-red LED light sources. Green and red light, which fall within the visible spectrum, offer the advantage of being less harmful to cells than UV or violet light, making them ideal for applications involving live cell encapsulation.
In this study, four cyanine dye-based photoinitiators—CZBIN, TDPABIN, Col-SH-CZ, and Col-SH-TD—were synthesized and evaluated for their potential in green-to-red LED photocuring. These initiators were designed to have strong absorption in the 400–600 nm range, making them suitable for use with green and red light sources. The two collagen-based macromolecule dyes, Col-SH-CZ and Col-SH-TD, demonstrated excellent cytocompatibility, making them particularly well-suited for cell-laden bioinks.
When incorporated into GelMA hydrogels, these novel photoinitiators facilitated efficient crosslinking under green and red light. Importantly, the crosslinking process was shown to have minimal impact on cell viability, with L929 cells encapsulated in the hydrogels achieving a viability rate of 90% after exposure to green light. This is a significant improvement over traditional UV-based systems, which often result in lower cell viability due to the cytotoxic effects of UV light.
The success of these novel photoinitiators in enabling cell encapsulation under green and red light highlights their potential for use in a wide range of bioprinting applications. Not only do they offer improved cytocompatibility, but they also allow for greater flexibility in the choice of light sources, making them a valuable addition to the toolkit of 3D bioprinting.
6.Applications and Future Directions
The development of biosafety photoinitiators for green-to-red LED crosslinking opens up exciting possibilities for the future of 3D bioprinting. By reducing the cytotoxicity associated with UV light, these new photoinitiators enable the fabrication of more functional, biocompatible tissue constructs that can be used in a variety of applications, ranging from drug testing to personalized tissue grafts.
One of the most promising applications of these technologies is in the field of regenerative medicine, where bioprinted tissues could be used to repair or replace damaged organs. The ability to print tissues with high cell viability is critical in this context, as the long-term success of the graft depends on the health and functionality of the cells within the printed tissue. The use of visible-light photoinitiators could also facilitate the incorporation of more complex biomaterials into bioinks, further enhancing the functionality of the printed tissues.
Looking ahead, there is potential to expand the range of visible-light photoinitiators available for 3D bioprinting. Researchers are already exploring the use of natural products, such as curcuminoids and flavonoids, as photoinitiators for visible-light-based bioprinting. These compounds, derived from natural sources, offer the dual benefit of being biocompatible and possessing strong light-absorbing properties in the blue-green range. Curcuminoids, for example, have been shown to facilitate free radical polymerization under blue-green LED light, making them promising candidates for future bioinks.
Moreover, advancements in dye-sensitized photoinitiation systems, where natural or synthetic dyes act as the primary light absorbers, could further expand the range of usable wavelengths. These systems, which operate based on photoinduced electron transfer (PET) mechanisms, offer flexibility in the choice of light sources and could enable the use of far-red or near-infrared light for bioprinting. This would be particularly advantageous for applications requiring deeper tissue penetration, as longer wavelengths of light are less prone to scattering and absorption by biological tissues.
Another promising avenue for future research lies in the development of hybrid photoinitiation systems that combine visible-light photoinitiators with other polymerization techniques, such as thermal or chemical crosslinking. By integrating multiple crosslinking mechanisms into a single bioink, it may be possible to achieve even greater control over the mechanical properties and biological compatibility of printed tissues. These hybrid systems could also enable bioprinting at multiple scales, allowing for the simultaneous fabrication of micro- and macrostructures within a single tissue construct.
7.Conclusion
3D bioprinting represents a revolutionary advancement in tissue engineering, with the potential to transform fields such as regenerative medicine, personalized healthcare, and drug testing. However, the success of bioprinting technologies depends heavily on the development of bioinks and photoinitiators that are both biocompatible and efficient in creating stable, functional tissue structures. Light-based 3D bioprinting, in particular, offers significant advantages in terms of precision and speed, but the reliance on UV and violet light poses serious challenges to cytocompatibility.
The introduction of green-to-red light photoinitiators, such as those discussed in this study, represents a crucial step forward in overcoming these challenges. These novel photoinitiators, designed to absorb in the visible spectrum, enable the safe and efficient crosslinking of hydrogels in the presence of living cells. Their high cytocompatibility and strong performance under green and red LED light offer exciting prospects for a wide range of bioprinting applications, from tissue scaffolds to organoids.
Future research in this area should continue to explore new classes of photoinitiators, including natural product-derived compounds and hybrid systems that combine multiple crosslinking mechanisms. By expanding the range of usable wavelengths and enhancing the functionality of bioinks, these innovations could significantly advance the field of 3D bioprinting and bring us closer to the realization of fully functional, bioprinted tissues and organs.
In summary, the development of biosafety photoinitiators for green-red light crosslinking in 3D bioprinting is a game-changer for tissue engineering. It offers the dual advantage of maintaining high cell viability while allowing for precise, light-based fabrication of complex tissue structures. As research progresses and new materials are introduced, the future of 3D bioprinting looks increasingly bright, with the potential to revolutionize medicine and create unprecedented possibilities for tissue repair and regeneration.